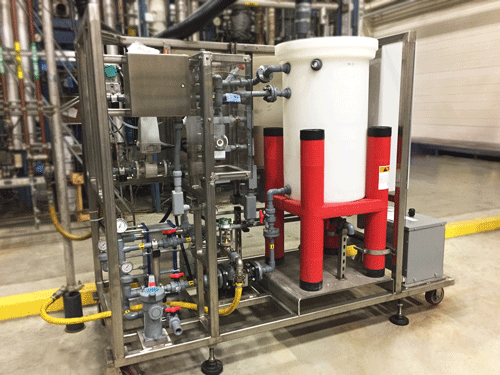
Per- and polyfluoroalkyl substances (PFAS) are a large range of synthetic fluorinated organic compounds that have been produced and widely used in industrial applications and consumer products since the 1930s. PFAS are commonly referred to as “forever chemicals” because they do not naturally break down. They are of concern due to their propensity to bioaccumulate.
An estimated 97% of Americans have detectable levels of PFAS in their blood, according to data from a National Health and Nutrition Examination Survey (NHANES) study as reported by the Centers for Disease Control and Prevention (CDC). In higher exposure cases, PFAS can be linked to cancer, liver problems, thyroid issues, birth defects, kidney disease, decreased immunity and infertility.
Acknowledging the risks associated with rising PFAS levels has led the United States Environmental Protection Agency (EPA) to move quickly to regulate these compounds’ presence. The EPA has set advisory health limits of four parts per trillion (ppt) for perfluorooctanoic acid (PFOA) and perfluorooctanesulfonic acid (PFOS) for municipal drinking water and combined hazard index limits for four compounds, GenZ, PFBS, PFNA and PFHxS.1
PFAS can be separated from contaminated water using several methods, such as ion-exchange (IX) resins, granular activated carbon (GAC), foam fractionation, reverse osmosis (RO), and aqueous electrostatic concentrator (AEC). However, these methods create a PFAS-contaminated medium or a concentrated PFAS effluent that requires additional treatment or safe disposal to avoid future environmental risk. Disposing of PFAS-contaminated materials in landfills is becoming unacceptable due to the risk of leachates reaching environmental waters, with destruction of collected PFAS currently deemed a more sustainable solution to end the contamination cycle.
PFAS destruction presents significant challenges due to the extremely high bond strength of the carbon-fluorine covalent bond (> 3.1 eV). Standard thermal waste incineration is a commercially available destruction method, but it risks reintroducing PFAS, particularly short-chain ones, into the atmosphere. Identifying proven, cost-effective and sustainable methods to destroy PFAS is paramount. Thermal solutions, including supercritical water oxidation (SWCO) and hydrothermal alkaline treatment (HALT), have been demonstrated to be effective in destroying PFAS waste, but commercial, practical and safety concerns remain on scaling these high-temperature batch processes.
Electrochemical Oxidation of PFAS
Since the first demonstrations of its destruction of PFAS in 2008 using boron-doped diamond electrodes (BDD), electrochemical oxidation (EOx) has been proposed as a single-stage remediation technology. However, EOx offers limited effectiveness in waters with PFAS concentration levels of < 1 ngL-1, where the transport of PFAS compounds to the electrode surface becomes more limited. Nonetheless, while other effective remediation and filtration techniques for drinking water have emerged, EOx has good potential as a final destruction technology for PFAS treatment steps. According to the May 2023 BlueTech Research Electrochemical Wastewater Treatment Insight Report, EOx is expected to be a dominant solution for some market verticals, especially PFAS.
Image 1 illustrates how the PFAS destruction rate is linear and only limited by the charge (electric current) reaching the electrode surface at concentrations above ~2,000 ngL-1. However, below this concentration range, EOx becomes increasingly inefficient because it is now limited by the rate of mass-transport to the electrode surface.
EOx should, therefore, be linked with remediation methods for PFAS-contaminated water streams that are themselves generating waste streams contaminated with high concentrations of PFAS. These remediation waste streams include depleted GAC, activated sludges, resin ion media or regenerated media that can have PFAS contamination in the range of 100 to 100,000s ngL-1, where EOx cells can operate under a regime with high oxidation efficiencies being guaranteed.
Compared to other PFAS destruction techniques—such as thermal incineration, HALT and SCWO—EOx operates at much lower temperatures and pressures, without the addition of hazardous chemicals. However, PFAS-contaminated waste streams are often a matrix of dissolved organics at the 10 to 3,000 mgL-1 range, with PFAS compounds in the 0.1 to 1000 µgL-1 range. Thus, the process economics are a complex trade-off of factors, including the generation rate of effluent, background organic load, relative concentrations, discharge limits, operating costs and scale. The treatment train must be optimized according to these factors for each particular application.
Electrode Material for EOx
Many different types of electrodes can be used for EOx, including a range of BDD subtypes and Magnéli-phase Ti4O7. The BDD subtypes vary in structure, phase purity, conductivity and corresponding electrochemical properties. Each type of electrode has been reported to have varying levels of suitability in terms of breaking down short-chain PFAS, long-term degradation in electrode effectiveness, generation of byproducts and the scaling requirements presented by large destruction plants. The main requirement for PFAS destruction is that the electrodes must have an extremely high oxidation potential, which can be characterized by the solvent window (Image 3).
BDD is a polycrystalline form of synthetic diamond. Since diamond is a semiconductor, the addition of ~0.5 to 2% boron-containing gas dopes the diamond as it grows, creating a polycrystalline p-type semiconductor with metal-like conductivity. BDD electrodes can be broadly divided into two types:
- thin-film BDD electrodes with a thin diamond layer, normally < 20 µm thick, coating a metallic substrate
- free-standing BDD electrodes that are solid diamond, normally > 300 µm thick, with no metal or silicon substrate (Image 2)
Thin-film BDD electrodes are made in hot-filament chemical vapor deposition (CVD) synthesis tools. Most commercial suppliers of thin-film BDD electrodes use refractory metals as the substrate (such as niobium, tantalum and tungsten) with the costs of these metals being a major factor in the total purchase price of commercially available thin-film BDD electrodes. Conversely, free-standing BDD electrodes are made in microwave CVD synthesis tools where the diamond is separated from a growth substrate, which is then reused.
Free-Standing BDD Electrodes
Free-standing BDD electrodes have the following advantages when compared to thin-film BDD electrodes:
- can be used in effluents at both extremes of pH levels and allow operation in strongly alkaline effluents, thereby keeping biproducts non-volatile
- are corrosion resistant because the electrode is wholly made of inert diamond
- have longer lifetimes, from three to 10 years or more, depending on effluent composition, due to the greater diamond layer thickness
- enable bipolar operation, by offering reversible anode and cathode
- have the widest solvent window (Image 3), operating at the highest current densities, up to 30,000 ampere per meter squared (A/m2), thus providing the highest oxidation potential (This is the most important for short-chain PFAS destruction.)
Short-Chain PFAS Destruction Using EOx With Free-Standing BDD Electrodes
The key challenge for PFAS destruction is the resident time for short-chain PFAS within range of the BDD electrodes. Experts suggest that PFOA breaks down in a multi-step process, sequentially producing shorter chain PFAS compounds.2
High current density conditions (> 2,000 Am-2) generate intense oxidation potential with much faster kinetics, which makes it more likely for breakdown to CO2 and fluorine ions to occur within the residence time as the molecules pass the electrode. As a result, the higher current density capability of free-standing BDD electrodes appears to be important in breaking the C-F bond in short-chain PFAS compounds.
Electrochemical oxidation has been used in the past to target low volume spent caustic waste streams. Some free-standing BDD electrode material can survive at the extremes of pH found in spent caustic waste streams. As the need for effective PFAS destruction technology has emerged, so has the need to test the effectiveness of a solution to destroy PFAS. Image 4 shows a schematic and 3D model of a pilot plant that conducted initial tests with synthetic effluents to prove that the BDD EOx solution could break PFAS down efficiently. The PFAS sample used for the tests consisted of
50 L DI water + 1.0 kg NaCl + 5 ml 40% PFOA (perfluorooctanoic sulfuric acid C8HF15O2). The electro-oxidation system was operated in a batch recirculation mode and samples were taken at predetermined time intervals.
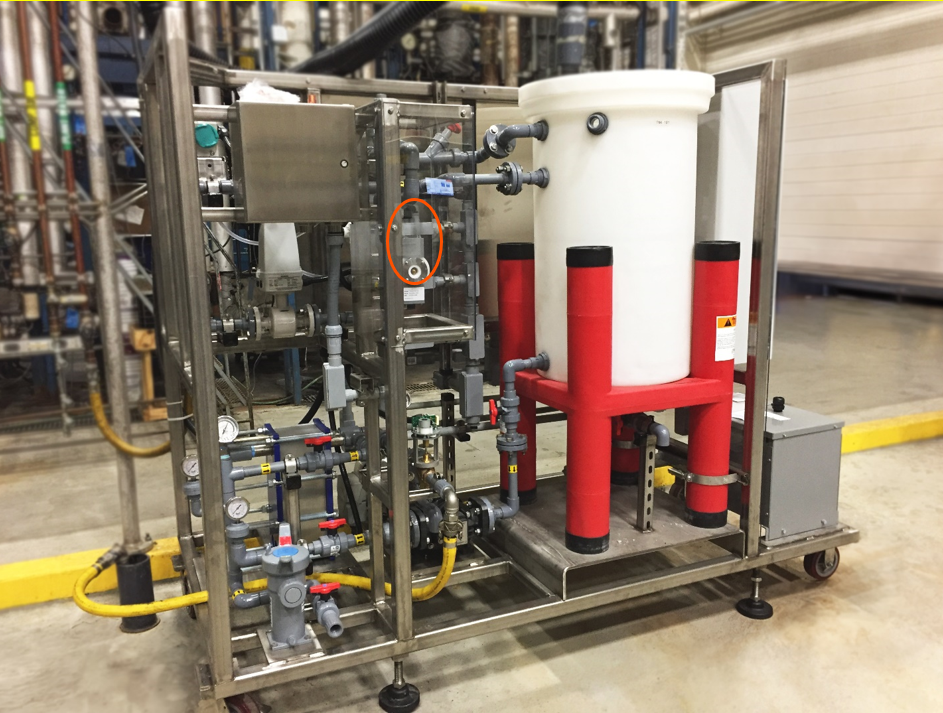
Using this equipment, the team tested PFOA and measured both its reduction as well as that of any resulting short-chain PFAS compounds. Image 5 shows the results of this trial using measurements performed via LM/MS analysis. The concentration of long-chain PFOA was reduced from an initial 100,000 µgL-1 to < 10 µgL-1: a greater than 10,000 times reduction, as indicated by the orange bars in Image 5. Short-chain PFAS compounds were created and then, over time, broken down as well, as indicated by the other bars in Image 5. Resulting short-chain concentrations were also < 10 µg/L for some of the compounds. After 34 kWh of energy, >99.8% of the original PFOA had been destroyed.
As international regulations around PFAS come into place and remediation technologies are developed, more attention is being placed on developing PFAS destruction solutions to be implemented alongside the remediation steps. Electrochemical oxidation processes offer significant advantages as a turnkey, low temperature solution to break down PFAS compounds.
Initial trials of EOx employing free-standing BDD electrodes to destroy PFAS show promising results. The extreme oxidation potential and high current densities (> 2,000 Am-2) that these electrodes create have been shown to destroy even the more recalcitrant short-chain PFAS compounds.
EOx technology has a major role to play in the destruction of PFAS and requires further testing of real-world samples, e.g. foam fractionated landfill leachate, which present a more complex matrix of short- and long-chain PFAS and recalcitrant dissolved organics.
References
epa.gov/sdwa/and-polyfluoroalkyl-substances-pfas
Chemical Engineering Journal 430 [2022] 132895